Since most of these diseases have a genetic component, next-generation sequencing is expected to help with genetic or genomic research.
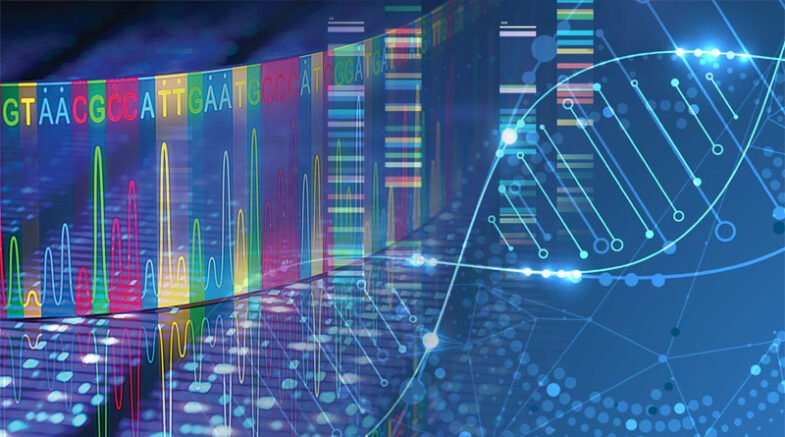
Along with increasing populations and newly invented livestock production methods, there has been a remarkable increase in pathogen transfer, whether between countries or continents. As well as diagnostics and cures, we are shifting to next-generation sequencing for the identification and treatment of illnesses.
Migration of people, animals, and daily goods, as well as climatic changes, have all had a significant impact on this factor, resulting in new epidemiological scenarios and threats to life. This new situation necessitates the development and application of a wide range of powerful novel diagnostic methods capable of rapidly detecting spreading pathogens with high specificity and sensitivity.
To meet this requirement, science has advanced in almost all measures, and as new diseases come into view, new methods are also being developed for their diagnostics.
The use of nanotechnology for cancer diagnostics
Nanotechnology has found its place in this world, occupying a unique position in cancer diagnostics. With the help of nanotechnology, a new generation of biosensors and medical imaging techniques with higher sensitivity and precision of recognition has been produced.
This technology revolves around the in vitro and in vivo diagnostic applications of nanoparticles and nanodevices that are likely to have an impact on the field in the future. Also, future developments may lead to the realization of multifunctional detection and treatment on nanoparticle platforms.
When it comes to cancer, finding it early and getting a better diagnosis is half the battle. Furthermore, tools that enable precise monitoring of patient response to therapy can optimize therapy and improve patient outcomes. Previously, when technology was not as advanced, the diagnosis and treatment of deadly diseases such as cancer was a nightmare for people, but now, along with innovations and the development of methods such as nanotechnology, these have greatly aided mankind.
The primary method for detecting cancer cells is the binding of nanoparticle probes conjugated with moieties (proteins, short peptides, antibodies, oligonucleotide parameters) to surface markers on cancer cells as well as those entering cells and detecting genetic content. For the detection of cancer cells, such as CTCs, capture or isolation is the first and most important stage.
Although the cell’s physical properties, such as size, deformability, and density, are sometimes used. The primary targets are the unique surface proteins on CTCs. Nanotechnology also makes it possible to send treatments directly to cancerous cells and tumors.
Clinicians can use these tools to deliver chemo therapies in a safe and effective manner, as well as radiotherapy and the next generation of immune and gene therapies to tumors. Nanotechnology tools can also help guide and improve the removal of tumors through surgery.
Next-generation sequencing to cure Neurological diseases
The phrase “neurological disease” covers a broad spectrum of conditions, many of which can’t be cured or are poorly understood, from early neurodegenerative disorders to late ones. Since most of these diseases have a genetic component, next-generation sequencing (NGS) is expected to help with genetic or genomic research.
It is considered difficult to translate the advancement of contemporary technology like next-generation sequencing into tangible benefits for patients and physicians, despite the fact that this is an exciting development. A clinical context would be ideal for a sequencing test that is quick, non-invasive, affordable, and has excellent specificity
However, before any next-generation sequencing diagnostic test can be used in the best way possible, there are a number of challenges and restrictions to consider. To successfully implement NGS diagnostic screening in clinics, it is important to correctly define the clinical phenotype, choose the best patients and clinical settings, maximize the test’s sensitivity and specificity, evaluate the availability of infrastructure and expertise, and think about economic, ethical, and legal issues.
Exome sequencing could change the way researchers look into rare Mendelian diseases. Aside from the technical benefits we’ve already talked about, one of the best things about this method is that it can find new mutations in an unknown gene in very small family trees.
In the recent past, this would have been impossible because genetic linkage or homozygosity mapping needed more than one person with the disease to find the disease locus. Lastly, exome sequencing can find mutations in genes that were not known before. This makes the symptoms of a genetic disorder more widespread. This is not possible with the candidate-gene-based diagnostic approach.
Exome sequencing is an exciting and very powerful method that is changing the way we study rare Mendelian diseases. It could be used to look into rare and common diseases, which could lead to the discovery of new mutations that cause diseases and alleles that are linked to diseases.
This would help us learn more about neurological diseases. While whole genome sequencing is likely to challenge its position at the forefront of neurogenetic research in the coming years, the basic science and clinical information learned from exome sequencing may prove extremely beneficial in shaping future research.
CRISPR as a diagnostic tool for inherited genetic variants
CRISPR, the abbreviation of Clustered Regularly Interspaced Short Palindromic Repeats, is the best-known gene editing system. The CRISPR uses the Cas9 protein paired with a guiding RNA; it’s engineered in such a way that it complements target DNA. When the target DNA is identified, Cas9 protein first cleaves the sequence at a defined site then permitting gene excision or insertion
The mechanism behind CRISPR diagnostics is similar to gene editing, in that it uses an engineered guide RNA. It uses different Cas proteins, namely Cas 12, Cas 13 and Cas 14. Further modifications of this protein generate fluorescent signals in the presence of a target nucleotide sequence, whether it be DNA or RNA.
As with most other molecular tests, CRISPR tests often need to go through an amplification step before they can be found. Polymerase chain reaction (PCR), a tried-and-true method that uses a thermocycler to multiply nucleotide sequences, is most often used to do this.
Two Nobel Prize winning commercial platforms, SHERLOCK and DETECTR have recently arisen, that used cas9 proteins in rapid in vitro identification of target sequences