As the highest-energy electromagnetic radiation in the Universe, gamma rays typically come from far away — from around black holes, supernovae and other extreme cosmic environments.
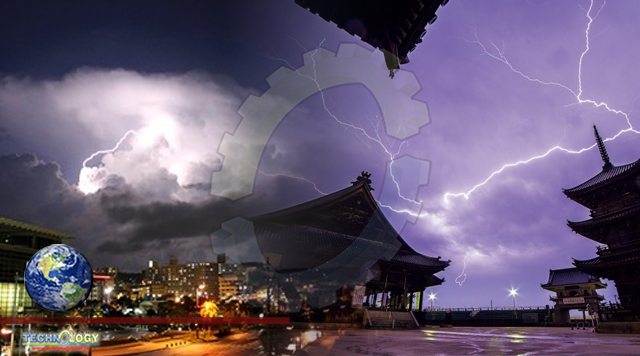
By Elizabeth Gibney
On top of Kanazawa Izumigaoka High School, the wind whips at researchers Teruaki Enoto and Yuuki Wada as they wrestle with a boxy instrument, trying to secure it to the roof.
A nearby weathervane swings ominously and clouds gather over distant mountains, all signs of the storm brewing in the direction of the Sea of Japan. This is exactly the kind of weather Wada and Enoto are hoping for. The device they are installing will spy on thunderstorms as they spit out gamma rays — a mysterious process that physicists are eager to understand.
As the highest-energy electromagnetic radiation in the Universe, gamma rays typically come from far away — from around black holes, supernovae and other extreme cosmic environments. They are often created by surges of electrons travelling at close to the speed of light.
But in the 1980s and 1990s, physicists discovered that clouds on Earth also emit invisible gamma rays: as short, intense millisecond bursts and as weaker, long-lingering glows.
Somehow, certain storms accelerate billions of electrons to close to the speed of light to produce these gamma rays. “The mystery is how this can occur in Earth’s atmosphere,” says Wada, a physicist with the Extreme Natural Phenomena RIKEN Hakubi Research Team in Saitama, Japan.
That question has brought him to a rooftop in a growing storm. Physicists want not only to understand this high-energy process, but also to use the radiation as a fresh lens for studying some fundamental questions about thunderstorms. There is even hope that the γ-rays might help atmospheric scientists to shed light on the centuries-old question of what initiates lightning.
But capturing these intense rays is not easy. Although satellites have spotted thousands of millisecond terrestrial gamma rays flashes (TGFs), those measurements can’t provide a close-enough view to reveal in detail the mechanism that produces them. Studying TGFs from Earth has previously proved difficult, and scientists have observed the longer-lasting glows at only a few locations.
Kanazawa is one of the best places to capture both glows and flashes. Located on the northwest side of Japan’s central Honshu island, the city regularly sees powerful thunderclouds that roll in from Siberia during winter and hover less than 1 kilometre above the ground.
Because the clouds are so low, radiation emitted by the storm can reach the ground, rather than getting absorbed by the atmosphere.
The group, led at the RIKEN Hakubi lab by Enoto, an astrophysicist, is making rapid progress in understanding these high-energy phenomena, says Joseph Dwyer, an atmospheric physicist at the University of New Hampshire in Durham. “These are some of the best researchers in the world in this field,” he says.
Around the globe, several ground-based groups are now looking at γ-rays from storms, including teams at large facilities designed to observe high-energy particles from outer space.
But by using a network of detectors, the small Japanese team has been one of the most successful in the world at spotting the phenomena — managing, on something of a shoestring budget, to detect ten TGFs and dozens of glows since 20151.
Wada and Enoto have big plans for their initiative, known as the Thundercloud Project. They are now expanding its work with the help of Japanese citizens.
This year, the team will create a network of around 50 detectors in schools, temples and homes that will allow them to catch more γ-rays, map the events and follow them throughout their life cycle, something that has never been tried before.
In Japan, the project is one of the first attempts in physics to harness research results through citizen science, says Yuko Ikkatai, a member of the initiative and a researcher in science communication at the Kavli Institute for the Physics and Mathematics of the Universe in Kashiwa.
The researchers eventually hope to place hundreds of small-scale detectors across the city. Focusing their efforts on Kanazawa was a gamble, says Enoto, but one that is already starting to pay off. “Now, I am glad,” he says.
Hidden flashes
Scientists first saw gamma rays glows coming from Earth in 1985, when a NASA jet carrying radiation detectors raced through a thunderstorm. It picked up weak emissions emanating from clouds before a lightning flash.
Then, in 1994, a probe designed to study the cosmos, NASA’s Compton Gamma Ray Observatory, detected TGFs coming from thunderclouds — much brighter bursts of gamma rays that last just hundreds of microseconds. It came as a surprise, says Dwyer, because the bursts stemmed from “the one place in the Universe everyone knew no γ-rays should be coming from” — Earth.
Elsewhere in the Universe, in energetic environments such as around black holes, beams of charged particles are accelerated to close to the speed of light and produce gamma rays when the particles collide with gas and dust.
On Earth, researchers now think something similar happens in certain types of thundercloud, in which strong electric fields accelerate electrons to extreme speeds. But physicists don’t know how big or intense the electric fields need to be to generate gamma rays — nor how to account for the sheer number of electrons a storm would need to make a TGF. “It’s a deep question at the moment,” says Enoto.
Observations by satellites, which routinely detect TGFs travelling up towards space, suggest that the flashes occur alongside lightning strikes. About 1 in every 1,000 strikes creates a TGF, meaning thousands of flashes occur around the planet each day.
But satellites are too far from the action to provide much detail. From a vantage point hundreds of kilometres above a storm, fast-moving satellites might capture only a handful of photons from each burst and struggle to pinpoint their position. Nor do they see the much weaker γ-glows.
Aeroplanes and balloons aren’t ideal platforms for studying the flashes either, because they can disrupt the natural phenomena and can be dangerous for researchers. Detectors on the ground provide a closer view than satellites do, but they are rarely close enough to storm clouds, so the γ-rays get absorbed well before they hit the ground.
“We really need close-up, detailed measurements of these things,” says Dwyer.
Citizen science
Enoto and his team can get close to storms in Kanazawa. In late 2019, before the coronavirus pandemic shut down international travel, they were gearing up for their winter campaign by reinstalling the first detector of the season.
In a taxi to the school, Enoto notes the bright red autumn leaves that draw visitors to Kanazawa’s famous public gardens. The city is “beautiful and old, with delicious food”, he says. But for researchers, it’s the city’s combination of frequent lightning and low-lying thunderstorms that are its best asset. “Thunderstorms here are special,” he says.
The Thundercloud Project began in 2015, when Enoto returned from a five-year stint in the United States and reignited a passion for the topic from a decade earlier.
As a PhD student in 2006, Enoto and physicist Harufumi Tsuchiya at RIKEN had installed detectors to explore strange noise-like signals picked up by γ-ray monitoring posts around nuclear power stations not far from Kanazawa, along the coast of the Sea of Japan. The detectors confirmed that such spikes came from winter thunderclouds passing overhead2.
When he returned in 2015, Enoto’s main field of study was cosmic sources of X-rays. But he never forgot the intriguing radiation much closer to home. Reunited with Tsuchiya, he embarked on an effort to build a fleet of detectors to explore these gamma rays signals around a wide area of Kanazawa, which is in Ishikawa prefecture, and in Niigata prefecture, to the northeast.
Crucial to their plans was making cheap, tabletop γ-ray detectors that they could install in dozens of sites. Wada joined the team and led the design of this compact device, which used a US$60 mini Raspberry Pi computer, alongside crystals of bismuth germanate, a material that lights up when it is hit by γ-ray photons.
Their experiments harness tools from high-energy physics that are rarely used by atmospheric scientists. “It’s a fusion of worlds,” says Wada.
At first, the project struggled to get a government research grant, in part because it falls between particle physics and atmospheric science. But the team was able to build a first generation of detectors with the help of a ¥1.6 million (US$15,000) donation from 150 supporters through the Japanese research-crowdfunding platform Academist.
In their first campaign, the researchers installed 16 detectors in Kanazawa and surrounding cities and, in 2017, made a major discovery. They observed a series of telltale γ-ray signals, which could only be caused by nuclear reactions in thunderstorms.
Their landmark findings proved that γ-rays could knock neutrons out of atoms in the air, making them radioactive. That finding confirmed the existence of a hypothesized process that produces some of the atmosphere’s supply of radioactive carbon-14, the isotope that researchers use in carbon-dating of ancient materials.
Now the team is expanding even further, in an effort to spot more γ-ray events and to better understand what causes them (see ‘Gamma-ray factory’). Kanazawa is an ideal location because it has a large inland plain that could host an array of detectors, allowing the researchers to track signals from travelling clouds.
Enoto has worked with a private company to design an even smaller, cheaper detector, which his team plans to distribute to citizen scientists around the city to install and operate.
Social network
Each yellow box, which is tagged with a GPS locator, is known as a Compact Gamma-ray Monitor or CoGaMo, the Japanese name for a type of small duck local to Kanazawa.
Alongside the existing network of detectors, the team has now placed ten CoGaMos in people’s gardens and homes. Most participants are friends of friends, with word having spread through a network of eager high-school teachers, says Ikkatai, who coordinates the citizen-science element of the project.
Later this year, after recruiting more participants in the citizen-science effort, the team hopes to have 50 detectors in operation, and 100 next year. The array would cover the region with detectors spaced about 1 kilometre apart. Although the full citizen-science project has yet to roll out, it already “gets more attention than my original area of X-ray astronomy”, says Enoto.
To recruit citizen scientists, the team has partnered with forecasting firm Weathernews, which already uses a fleet of volunteers to take and submit photos that improve the company’s live weather reports.
Members of the public will be able to use the Weathernews web system to upload photos during storms, and people with a CoGaMo detector will receive automatic prompts to do so during γ-ray glows.
Such data will be invaluable, says Enoto, revealing features such as cloud structures, geometry, size and colour during the events. “The big question I want to know is, what kind of thunderstorms can generate γ-rays?” he says.
“We do not know what the difference is between the standard type of thunderstorm to the strange type that shows γ-rays.”
Citizen photos will give a more complete picture of a thundercloud’s features when it produces γ-rays than would be possible with just radar or other conventional methods, says Vanna Chmielewski, an atmospheric scientist at the US National Severe Storms Laboratory and the University of Oklahoma, both in Norman. “The citizen-science part is honestly one of the things about the work that I am the most excited about,” she says.
Enoto’s team wants to use its detectors to understand the size of γ-ray emitting regions, and how they vary in time and space and with a cloud’s movement.
One of the keys to the group’s success is that “they’ve been able to put these things all over the place”, says physicist David Smith at the University of California, Santa Cruz, who has been studying high-energy phenomena in storms since the early 2000s.
Smith and his colleagues have installed a single detector system on Japanese soil, also in the Kanazawa region, in collaboration with physicist Masashi Kamogawa at the University of Shizuoka.
They have seen just two γ-ray flashes so far. But Smith’s group now hopes to emulate Enoto’s approach by making smaller, cheaper and less-sensitive versions that could be produced by the hundreds and distributed widely — perhaps in collaboration with the RIKEN team. “That’s my dream,” he says.
Inside the cloud
Physicists understand the basic process behind glows and TGFs, but many questions remain. One key player is the strong electric field in thunderclouds. The field forms when rising streams of air carry ice crystals upwards past falling hail — friction between the two creates separate pools of negatively and positively charged particles in different parts of the cloud.
Those fields are natural particle accelerators. If a very high-energy electron (perhaps generated by a cosmic ray from outer space) enters the cloud’s electric field, it can overcome the friction of air to accelerate to close to the speed of light4.
When that electron hits an air atom, it releases the kind of γ-ray seen in glows and flashes, in a process known as bremsstrahlung radiation. Electrons multiply because each collision can knock further electrons out of the atoms in a chain reaction, creating an avalanche of particles and a flood of γ-rays.
In γ-ray glows, this particle cascade happens at a slow rate; in a TGF, it is explosive. Smith says it’s like the difference between a nuclear reaction at a power plant and what happens in a fission bomb.
The mystery is in the details. The accelerator mechanism that researchers know about can’t produce enough electrons to generate the TGF, meaning that some other process must also be at play.
The link between γ-ray phenomena and lightning also remains murky. The flashes appear at the start of lightning strikes, and are possibly triggered by them, whereas glows can start minutes before lightning happens.
Enoto hopes that his project’s data will help to improve understanding of these natural particle accelerators. His detectors can see TGFs, but the flashes are so bright that they saturate the instruments, so researchers cannot yet study them in great detail. If the current field trial is successful, Wada hopes to install extra detectors in the CoGaMos that could better capture the TGFs, helping researchers to decide between rival ideas about their origins.
Researchers have posed two main possibilities. One hypothesis suggests that the electrons are released at the tip of lightning ‘leaders’ — the narrow conductive channels that occur before the larger visible lightning current. According to this idea, the extreme electric field at the tip of a leader can ionize the air, creating trillions of ‘seed’ electrons.
Another proposed mechanism, which Dwyer has termed dark lightning, says that the avalanche process itself would result in many more electrons than initially proposed, because some of the γ-rays created by high-energy electrons would trigger whole new cascades — an avalanche of avalanches.
For now, the real strength of the Japanese array will be in exploring glows, the flashes’ baby cousins. The team now sees as many as 20 glows a year in Kanazawa, and uses data from radio-frequency receivers to chart lightning — working with collaborators who detect the strength and position of strikes using radio emissions, as well as measuring precipitation and other conditions using radar.
The team wants to use its array to track glows as they drift for kilometres, to learn about the lifetime of the strong electric fields in storms that create them, including how particle acceleration starts, how it develops and what stops it.
In 2019, the team became the first to definitively show a growing glow suddenly terminating with a γ-ray flash, as well as lightning6. “It was a beautiful result,” says Smith.
To Enoto, this is a hint that the flow of high-energy electrons that causes the glow could trigger lightning and its associated TGF, but the team needs many more observations to conclude that. The idea is an “exciting possibility”, he says.
Lightning source
What triggers lightning is one of the biggest mysteries in atmospheric science. “Benjamin Franklin studied lightning centuries ago, but there’s still so much we don’t know about how it forms and how it develops,” says Chmielewski.
The problem is that the electric fields seen so far in storms seem too weak to ionize atoms in the air — the process that allows electric current in the form of lightning to connect the two regions of separated charge.
Physicist Ashot Chilingarian says there is indeed evidence that the avalanche of electrons involved could open the path for lightning leaders. His team at the mountain-top Aragats Cosmic Ray Research Station of Yerevan Physics Institute in Armenia is the only other in the world to have seen large numbers of glows, witnessing hundreds of events.
They refer to the glows by a more general term — thunderstorm ground enhancements — because their detectors also pick up the electrons and other knock-on particles.
One reason that lightning has remained a mystery for so long is that it’s hard to safely study the electric fields inside storm clouds. Whether glows act as a trigger or not, they are an important tool for studying lightning because they reveal the conditions inside a storm, says Dwyer. The gamma rays tell researchers how long an electric field persists, as well as how strong it is. “These are really hard measurements to make directly,” says Dwyer.
Moreover, glows often terminate in lightning, which eventually pulls the plug on the accelerator by dissipating the electric field. If the team can tease apart the conditions that cause glows to result in lightning, Wada also hopes it might be possible to use the γ-ray signal to predict strikes minutes before they hit, potentially saving lives and protecting property.
Understanding how common TGFs are, as well as what kind of lightning tends to trigger them, is important for another reason, says Smith: they can be dangerous in some situations. Glows are too weak to cause an issue, and by the time TGFs reach the ground they are usually harmless. But up close, the flashes are much more potent.
If one hit a plane, for example, “in the worst case scenario, you could have people walking off the plane with visible signs of radiation sickness”, says Smith. It’s reassuring that this has never been seen, he says.
And it might not be a problem for aircraft because they often trigger lightning, which means they might do so before the electric field becomes strong enough to make a gamma rays flash. But he wonders whether people on planes could receive smaller doses that go undetected. “It might result in 2 or 3 cases of cancer 20 years down the line and you would never know. But it’s important to know,” he says.
Enoto’s team is now considering putting CoGaMos on Japanese passenger aircraft to see whether they can detect such invisible radiation from the air. And they’ve got the bug for using small detectors in all kinds of location: starting in 2022, the team plans to put shoebox-sized CoGaMo-like detectors on tiny satellites called cubesats.
This will enable the study of X-ray emissions from cosmic sources that are so bright they saturate billion-dollar telescopes such as NASA’s Chandra X-ray Observatory.
Back at Kanazawa Izumigaoka High School, with the detector successfully secured on the roof, Wada, Enoto and their colleagues retreat to a warm classroom, where a dozen eager students soon gather.
Initially shy, the students eventually pepper with Enoto with questions about the detectors, radiation and the local storms — which they had no idea could be so fruitful. Wada is delighted. “We want to make this research open to everybody,” he says.
Originally published at Nature