Cold Plasma Can Be Used To Decontaminate Products That Have External Microorganisms. It Can Also Be Used To Disinfect Surfaces.
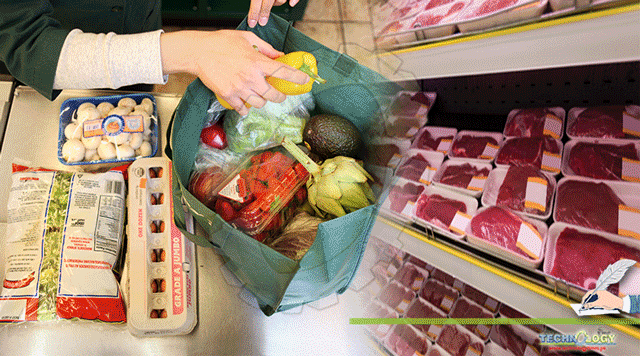
Introduction
Minimally processed foods are one of the most important growth sectors in the food industry. This growing demand for healthy and convenient foods with fresh properties has been accompanied by concerns about the effectiveness of available sanitation methods to adequately treat foodborne illness. In fact, chemical disinfectants do not provide effective microbial reduction, but are not perceived negatively by consumers, they are dangerous to human health and harmful to the environment, and traditional heat treatments can negatively affect the physical, nutritional or bioactive properties of these perishable foods. For this reason, the industry is investigating alternative non-thermal physical technologies, namely innovative packaging systems, radiation, pulsed light, high power ultrasound, cold plasma, high hydrostatic pressure and carbon dioxide in the dense phase, as well as possible combinations between these. or with other conservation factors.
Pulsed Light:
Pulsed light (PL) is a fast and environmentally friendly emerging technology for decontaminating food surfaces by inactivating microorganisms with intense broad spectrum pulses rich in UV-C light. PL works with xenon lamps, which can generate intense and short-duration pulses of broad “white light”, from ultraviolet wavelengths of 200 nm to infrared wavelengths of 1000 nm with maximum emissions between 400 and 500 nm. Power is increased many times by adding energy stored in a high-performance capacitor for relatively long periods of time (fractions of a second) and released over a short period (millionths or thousandths of a second), generating several high-energy pulses per second. Pulsed light (PL) consists of a sequence of extremely short and powerful pulses of white light with a broad spectrum, containing about 54% ultraviolet (UV) light, 20% infrared and 26% visible light radiation. Unlike conventional or continuous UV light, it is considered an effective alternative to microbial inactivation during food processing, production and handling due to its high penetrating power and the rapid dissipation of high energy pulses. In a typical PL emission process, high voltage is used to generate an inert gas, e.g. B. Xenon to excite from a ground state to an excited state. The xenon then returns to its ground state, the energy is released in photons and absorbed by food molecules, leading to a series of photothermic, photophysical and photochemical reactions in the food material. This phenomenon increases the instantaneous energy intensity, which contributes to the inactivation of pathogenic and decomposing microbial cells. These photographic effects are responsible for how PL interacts with food molecules, with benefits such as lower energy consumption, less time consumption, absence of waste and ease of adaptation. Furthermore, the negative effects on food quality in terms of nutritional and organoleptic properties may be limited since there is no significant increase in temperature during treatment. This novel technique is proposed for the decontamination of solid and liquid foods, containers, medical products, packaging and processing equipment for the food, medical and pharmaceutical industries. One of the properties of photoreactive food biomolecules is the presence of chromophores in the molecule, and extensive exposure of food to ultraviolet light leads to depolymerization of starch, peroxidation of unsaturated fatty acids, and the formation of insoluble complexes. Proteins are prone to photoreactions because they contain efficient chromophores, e.g. Ex. B. prosthetic groups and amino acid chains. Absorption of light by protein chromophores at high energy levels leads to side chain oxidation, protein crosslinking and aggregation, insoluble protein formation, and backbone fragmentation, and as a result can lead to a change in binding of IgE to allergens. The efficiency of inactivation of PL depends on the intensity (measured in J cm-2) and the number of pulses delivered. The UV-C part of the spectrum of light emitted by the flash lamp (200-280 nm) is the most lethal and therefore the most important for microbial inactivation. As a result, cells are inactivated by photochemical damage to DNA, protein denaturation, agglutination of cytoplasmic content leading to destruction of cell membranes, and other photothermal and photophysical effects. Therefore, the significant and rapid microbial inactivation in short-term treatments, the lack of residual compounds and a great flexibility are some of the advantages of this technology. Furthermore, recent findings show that PL kills yeast through a mechanical or multiple impact process that affects the permeability of the cell membrane, as well as the stability and functionality of DNA and macromolecules, depending on the dose used. Even so, the composition of the food affects the effectiveness of this technology. The presence of proteins and oil reduces the decontamination effect as they absorb the effective wavelengths. On the other hand, carbohydrate-rich foods like fruits and vegetables seem to be more suitable for PL treatments. However, some studies have examined the effects of PL on the physiological and quality parameters of fruits and vegetables. The inactivation of microorganisms naturally present on surfaces of fruits and vegetables or inoculated by PL has been achieved and tested in recent years.
High hydrostatic pressure
High Hydrostatic Pressure (HHP) processing is a relatively new and promising non-thermal food processing technology that exposes liquid or solid foods, with or without packaging, to pressures between 50 and 1000 MPa. It is an excellent alternative to heat treatments to destroy foodborne pathogens and inactivate enzymes. High Pressure Processing (HPP) is a non-thermal technology that offers excellent opportunities for food safety through microbial decontamination of food and shelf life extension with minimal changes in sensory and nutritional profile. There are three key parameters in HPP (i.e. temperature, pressure, and exposure time) that allow adaptability of the process design. In a HHP process, the food product to be treated is placed in a pressure vessel that can maintain the required pressure and is immersed in a liquid (generally water) that acts as a pressure transmitting medium. The pressure is transmitted uniformly (isostatically) and almost instantly over the entire food sample. Therefore, unlike heat treatment, the time required for print processing is independent of the shape or size of the food. Furthermore, there are only slight fluctuations in temperature with increasing pressure (the temperature increases by approximately 3 ° C per 100 MPa, depending on the composition of the feed). These special characteristics prevent the food from warping or heating, which would change its organoleptic or nutritional properties. In general, low molecular weight compounds are only marginally affected by HHP, since covalent bond breaking does not occur at pressures below 2 GPa. due to its very low compressibility. Therefore, the primary structure of volatile compounds, pigments, vitamins, and other compounds associated with the sensory, nutritional, and health-promoting aspects of food are less or rarely affected. This effect is important for fruits and vegetables when they are rich in bioactive ingredients (including vitamins) and pigments. Due to the poor compressibility of covalent bonds, flavors, vitamins and minerals are rarely influenced by HPP, so pressure treatment is mostly ineffective: Valent bonds such as hydrophobic, hydrogen and hydrophobic bonds Ionic and the effect of high pressure are mainly related to cleavage. With respect to proteins, since the primary structure of proteins consists of a sequence of amino acids linked by covalent bonds, they are not affected by very high pressures. Whereas secondary protein structures, consisting of a polypeptide chain that forms α-helixes or β-sheets / strands through intra- or intermolecular hydrogen bonds, are influenced by HPP due to hydrogen bond fragmentation, metal ion bonding and salt bridges. HPP also has a huge impact on the tertiary and quaternary structures of the protein, as they are maintained by non-covalent bonds. In general, a triple effect pathway has been observed when pressure is applied to protein molecules, depending on the amplitude of the applied pressure: First, the primary and secondary bonds are subject to reversible distortions using low pressure. Although the volume change in the system due to compression of primary bonds is not considered relevant, compression due to hydrogen bonds leads to conformational changes that can reduce the dimension of the internal cavities in the biomolecule. Changes in tertiary structure and other intermolecular interactions occur at 200 MPa due to an increase in the molecular surface area, which in the monomer state leans towards the dense hydration layer, and due to voids in the internal surface of the aggregates due to improper packaging. Quaternary structures dissociate at pressure levels of 150 to 200 MPa. A further increase in pressure to 500 MPa could unfold the protein, which is evidenced by a disordered structure that is typical of biomacromolecules. At this pressure, the unfolding of proteins allows the SH groups to be exposed and the number of unmasked SH groups to decrease as the treatment progresses, probably due to the formation of disulfide bonds by oxidation, especially at alkaline pH where thiolate anions they are more reactive.
IRRADIATION
Food irradiation is a technology that can be safely used to reduce food loss due to spoilage and control contamination that causes illness and death. When irradiating food, beams of electrons, gamma rays, or X-rays with radiant energy are used to rid the food of harmful microorganisms, insects, fungi and other pests and delay spoilage. It does not make food radioactive. Irradiation kills pathogens and it can no longer reproduce. There are several processes that are collectively known as food irradiation. Each process has an optimal dose of ionizing energy (radiation), depending on the desired effect. The radiation dose is measured in gray (Gy). A gray is a unit of energy that corresponds to 1 Joule per kilogram. This unit of measure is based on the metric system. Thus, 1 kilogray (kGy) corresponds to 1000 shades of gray (Gy). Irradiation involves exposing food materials to electrons or X-rays generated by an electron accelerating machine, or γ-rays generated by a radioisotope source such as cobalt 60 or cesium 137. In general, an intermediate dose (1-10 kGy) to achieve pasteurization of perishable products such as fruits and vegetables, while higher doses in the range of 10 to 50 kGy are used to sterilize low-moisture foods such as condiments. Γ rays easily penetrate materials and are typically used during bulk processing, while electron beams are used for surface irradiation. Although γ-irradiation offers numerous benefits for food safety, the technology can induce changes in the functional integrity of biomolecules. In proteins, exposure to γ-rays accelerates cross-linking, unfolding, fragmentation, and the creation of new reactive groups, leading to changes in protein structure. These changes are caused by the breaking of covalent bonds, either directly by photons or indirectly by reactive oxygen species (ROS). Gamma rays, The rays used in food processing are obtained from large sources of 60Co radionuclides. This type of radiation is essentially monoenergetic (60Co simultaneously emits two photons per decay with energies of 1.17 and 1.33 MeV). With analytical techniques such as the point kernel or the Monte Carlo method, it is possible to calculate the dose distribution in irradiated food, even when using very complicated source geometries, such as spread plate sources. The resulting distribution of depth dose in food generally resembles an approximately exponential curve. The electrons emitted by accelerators have fairly narrow spectral energy limits (generally less than ± 10% of the nominal energy). The energy of the electrons reaching the product is optionally further controlled by the bending magnets of the beam handling system. The range of an electron in a medium is limited (in contrast to that of photons) and is closely related to its energy. The effectiveness of ionizing radiation food processing depends on the correct delivery of the absorbed dose and its reliable measurement. Food packed in boxes or crates is placed on conveyor belts and transported to the heart of the irradiation machine, where it is exposed to the radiation source. X-ray generation is energy intensive and inefficient because only about 4 to 6% of total electrons generate X-rays. Electron beam emitters can clean packaged food at the end of food processing production lines . High-energy waves pass through food and stimulate electrons in food, as well as pests or pathogens. When electrons absorb enough energy, they break away from their atoms and leave positively charged centers. The molecular structure is altered by irradiation; kills or reduces the amount of bacteria and yeast; slows mold growth; and sterilizes or kills parasites, insects, eggs and larvae. Absorbed radiation values are currently measured in kilo gray (kGy). Irradiation can make meat and meat products safe by killing various pathogens and parasites. This improves the shelf life and shelf life of foods by better maintaining the quality of nutrients.
Pulsed electric field (PEF)
Pulsed Electric Field (PEF) is an excellent non-thermal food preservation technique that uses short pulses of electricity to permeabilize and disintegrate microbial cells, accompanied by minimal changes in the quality characteristics of the food product. Typically, high voltage pulses of around 20-80 kV are applied to the electrically conductive food placed between the generator electrodes. The process consists of placing the food material between two electrodes and passing pulses with a high intensity electric field (1-50 kV / cm). Since legumes are applied for a short period of time (2 μs to 1 ms), the negative impact on food quality due to heat treatment is greatly reduced. Non-thermal processing is an important technology that is widely used in the food industry to extend shelf life and ensure food safety at low processing costs. It is used primarily for microbial decontamination. PEF can also be used to inactivate enzymes, improve mass transport during drying, and possibly reduce allergens. Among all the emerging non-thermal technologies, high intensity pulsed electric fields (PEF) are one of the most attractive technologies due to their short treatment times and reduced heating effects compared to other technologies. When processing High Intensity Pulsed Electric Fields (PEF), high voltage pulses (typically 20 to 80 kV / cm) are applied to food that are placed between two electrodes. The PEF treatment is carried out at room temperature, sub-room temperature or slightly above room temperature for less than 1 second, and energy loss due to heating of food is minimized. In terms of food quality properties, PEF technology is considered superior to traditional heat treatment of food as it avoids or greatly reduces harmful changes in the sensory and physical properties of food. The application of an electric field leads to cell death due to the formation of pores (electroporation) in the bacterial cell membrane without affecting the enzymes or proteins present in food. The membranes of cells treated with PEF become permeable to small molecules; Permeation leads to swelling and eventually to breakdown of the cell membrane. A 400 V / cm PEF treatment was applied. PEF pretreatment increased juice yield by 67-75% compared to the untreated control sample. PEF samples pretreated during air drying showed a reduction in drying time of approximately 25% compared to control samples. The total specific energy consumption was 3.0 kJ / kg and the increase in temperature due to the PEF treatment was less than 1 ° C.
Ultrasonication
Ultrasonic treatment is a versatile technology with wide food applications including drying, sterilization, enzyme inactivation, extraction, filtration, homogenization, tenderizing of meat, etc. It is widely welcomed as being safe, environmentally friendly with reduced processing time and inexpensive cost compared to traditional techniques. Ultrasound (USA) is an environmentally friendly processing technology that holds great promise in the food industry due to its potential to inactivate microorganisms on fruit and vegetable surfaces. The American treatment is simple, relatively cheap, and energy efficient. It also offers advantages in terms of cost, productivity and selectivity, better processing time, better quality and fewer physical risks. High-power ultrasonic waves can induce modifications in materials by physically dissolving or accelerating certain chemical reactions by accumulating pressure and temperature gradients in the material in which the waves travel. Interestingly, the waves generated can be absorbed, resulting in a multitude of changes in the characteristic nature of the food. These effects are caused by the acoustic cavitation that is generated during sonication, that is, H. Through the creation, multiplication and sudden collapse of the bubbles present in the material. As a result, high pressure and local heating build up. The American treatment is based on the energy generated by sound waves with a frequency that exceeds the limit of human hearing. In relation to the American system, it consists of a power generator (power source), a converter (to convert electrical energy into American frequencies) and a coupler or emitter (to emit the American waves from the converter to the medium). The US band can be divided into low power (high frequencies) and high power (low frequencies) ultrasound. The former covers frequencies above 1 MHz and has non-destructive effects on the properties of the food they pass through. On the other hand, low frequencies between 18 kHz and 100 kHz induce mechanical, physical and chemical changes, eg. Eg B. physical alterations that support the inactivation mechanism on the food surface. In food preservation, high-performance ultrasound is used to inactivate microorganisms. The antimicrobial effects are attributed to the phenomenon of intracellular acoustic cavitation, which is characterized by the formation, growth and implosion of small bubbles generated by American waves. These bubbles traverse the biological structure and promote a series of compression and dilution (expansion) cycles that lead to local energy generation (hot spots). Two phenomena of cavitation can occur, stable cavitation and transient cavitation. Hot spots caused by bubble implosion events, which are characterized by high temperature (up to 5500 ° C) and high pressure (up to 1000 MPa), occur in very short periods of time (μs) and lead to release reactive species. (eg hydroxyl radicals) due to dissociation of the vapor trapped in the bubbles. In addition to the physical effect that is responsible for microbial cell death, the resulting reactive species also contribute to microbial inactivation. In general, gram-positive bacteria are more resistant than gram-negative bacteria due to their thicker cell walls and probably due to a tightly adhering peptidoglycan layer on gram-positive cells. Cocci are also tougher than rod-shaped bacteria, and larger cells are more sensitive than smaller ones. Furthermore, spores appear to be more resistant than vegetative forms, whereas enzymes are said to be inactivated by ultrasound due to a depolymerization effect. The pH also influences the effectiveness of decontamination, as the inactivation rate increases at a lower pH. Recent studies have shown that the amplitude of the ultrasonic waves, the exposure time, the treatment temperature, and the volume and composition of food can influence the properties of fresh fruits and vegetables differently. Fresh fruits were treated at a frequency of 0, 25, 28, 40 or 59 kHz (operating at 350 W power) at 20 ° C for 10 minutes and then stored at 5 ° C for 8 days. It was found that 40 kHz significantly reduced the incidence of caries and the microbial population and inhibited the decrease in firmness and maintained significantly higher levels of TSS, titratable acid and vitamin C.
Cold plasma
Cold Plasma (CP) is a new and environmentally friendly food preservation (decontamination) technology that has the potential to be applied to a wide variety of foods. It is so new that the terminology has evolved and has only been used on a very small scale. In fact, various PC technologies have been developed for food processing, as well as technologies for their production. Non-thermal plasma is made up of quasi-neutral ionized gases, which dissociate through an energy supply. These ionized gases include particles such as photons, free electrons, positive and negative ions, atoms in their ground or excited state, and free radicals, which in combination have the ability to inactivate microorganisms on the surface of food. The gas to be ionized can be air, O2 or N2 or a mixture that contains a certain proportion of noble gases (Ar, He or Ne). Heat, electricity, laser light, and radiation are used as energy sources. Plasmas can be generated at atmospheric pressure and close to room temperature, allowing non-thermal treatment conditions. As a cloud of active particles, the recombination process of this species creates energy that is retained in the plasma for a certain period of time and then released as visible and ultraviolet light. Active particles in plasma can react with the food substrate and release stored energy to target bacteria or viruses. Therefore, microbial inactivation can take place through the chemical interaction of radicals, reactive species or charged particles with cell membranes, through damage to the membranes and internal components of cells by UV radiation or by breakage of DNA strands also by UV light. However, the antimicrobial mechanisms of action depend on the source and type of plasma generated, whose total energy varies with the gas used, the density and the temperature. PC systems can be divided into three categories based on where the treated food is placed in relation to the PC source and the type of PC chemistry. The first category is the remote treatment system, where the food product is placed some distance from the generation source, which is treated with plasma rich in secondary chemical species. The second is known as a direct treatment system, in which the product is placed relatively close to the source of generation and treated with active plasma. and in the third category, electrode contact systems, the product is in the CP generation area. The effective treatment time varies from less than 3 s to 300 s, depending on the physical (food form) and chemical properties of the food and the processing conditions. The effectiveness of decontamination also depends on the type of microorganism, the inactivation medium, the number and physiological state of the cells, and the operating gas flow and mixture. Cold plasma can be used to decontaminate products that have external microorganisms. Unlike light (eg UV light decontamination), plasma flows around objects, which means there are no shadow effects to ensure that all parts of a product are treated. There is currently no technology available to decontaminate soft surfaces for products such as cut vegetables and fresh meat. Cold plasma could be used for this purpose. Cold plasma can also be used to disinfect surfaces prior to packaging or as part of the packaging process. Atmospheric plasmas containing a high proportion of bactericidal molecules (> 100 ppm ozone, nitrogen oxides, peroxides, etc.) are generated with minimal power under ambient temperature conditions in seconds or minutes with little or no heating of the product. APT can only produce bactericidal molecules very efficiently from air if the product temperature rises below 5 ° C. These unique flexibility and processing capabilities are driving the technology into new markets and applications. Atmospheric plasma offers a number of advantages over existing food safety technologies, including the following: 1) It is a dry process; 2) easily adapts to a food production environment; 3) requires very little energy; 4) the reactive gas species return to the original gas within minutes to hours after treatment, and 5) short treatment times are required.
Dense phase carbon dioxide
Recently, special attention has been paid to dense phase carbon dioxide (DP-CO2), a collective term for liquid, high pressure or supercritical CO2, as an alternative non-thermal treatment for the preservation of fresh fruits and vegetables among other foods. . It uses pressures combined with CO2 to kill microorganisms as a food preservation agent. DP-CO2 describes the phases of matter that remain liquid, but are dense relative to gaseous CO2. CO2 is inert, non-toxic, non-flammable, inexpensive, readily available in high purity, and leaves no residue, which is generally recognized as safe. This gas is also a natural component of many foods. When operated under supercritical conditions (7.4 MPa and 31.1 ° C), it has very good mass transfer properties (low viscosity and zero surface tension) and ensures efficient penetration into solid matrices. At the end of the treatment, it can be easily removed from the solute by simple expansion to ambient pressure. Microbial inactivation can occur by physical alteration due to rapid depressurization and expansion of CO2 in cells. CO2 increases the fluidity of the membrane due to a loss of order in the lipid chains, which leads to higher permeability. The dissolution of CO2 in the aqueous component of foods leads to carbonic acid, which reduces the extracellular pH and consequently inhibits many metabolic systems of the cell. Gram positive bacteria are more difficult to inactivate than gram negative ones, mainly due to the thickness and composition of the cell wall. One of the first food applications was the treatment of whole fruits to inhibit the growth of mold. Recently published data has shown that this technology can effectively inactivate microorganisms and enzymes in minimally processed fruits and vegetables. It is considered a promising and sustainable technology for preserving a wide variety of foods. However, studies on the effects of this technology on food quality parameters are still limited. High Pressure Carbon Dioxide (HPCD) is another upcoming treatment that is being widely used as a non-thermal technique for pasteurizing food. The process is not only environmentally friendly due to the non-toxic nature of carbon dioxide, it also involves the use of a lower CO2 pressure than HPP. The use of lower pressures makes this technique an energy saving process. Furthermore, the creation of carbonic acid (H2CO3) in the water contained in food causes a decrease in the pH of food products, which improves the penetration of CO2.