Cutting the cord at home—replacing , LED-Lit Future for Ocean Sciences traditional cable television service with alternatives like streaming video—is an increasingly common trend these days. In oceanography, scientists are seeing their own version of this trend, one that might more accurately be called “cutting the tether.” Both trends have been matched—and aided—by the rapid growth of light-emitting diode (LED) technology and applications.
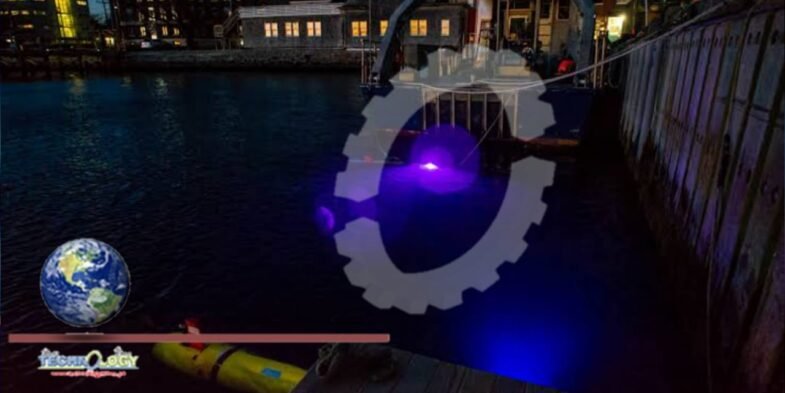
Recently, there has been a push in the oceanographic community to replace hard-wired, fiber-optic communication tethers connected to instruments with wireless, through-water communications. Think Wi-Fi for the ocean. For example, in 2019, untethered, wireless communications allowed then Seychelles president Danny Faure to address his constituents and raise awareness about ocean health from a submersible vehicle 124 meters below the sea surface, an event that was livestreamed to thousands around the world. The core technology that made this livestream possible comprised high-powered, blue LEDs that package and rapidly transmit data through the water
The foundational technology of LEDs has been around for more than a century, but only in the past decade or so have LEDs become transformative to society.
In 2010, LEDs accounted for 1% of the global lighting market; by 2020, this share had risen to 55%. This rapid growth has profoundly reshaped the industry, even contributing to the decision by General Electric, long known as the leading innovator in lighting, to sell its lighting division in 2020. At some point this decade, the entire global lighting market is expected to shift to LEDs, with the LED lighting market rising from roughly $60 billion in 2021 to an estimated $135 billion in 2028.
The transition from incandescent bulbs to LEDs has been driven by both policy changes and consumer demand. In the United States, for example, the Energy Independence and Security Act passed in 2007 mandated that many household lightbulbs become 25% more efficient than incandescent bulbs. Similar polices have been adopted by the European Union and China.
These policy changes left consumers with two options: compact fluorescent lamps (CFLs) or LEDs. LEDs are more than 40% more energy efficient and last 3–7 times longer than CFLs, and as LED bulb prices have dropped, consumers have increasingly opted for them.
Diagram showing relative magnitude of impacts of different lighting sources over their life cycles on natural re-sources, air, soil, and water.
Shown here are the relative magnitudes of impacts of different lighting sources over their life cycles on natural resources, air, soil, and water. The impacts of compact fluorescent (red) and LED (green and purple) bulbs are substantially less than those of incandescent bulbs (blue). Credit: U.S. Department of Energy
Although the choice for consumers has been relatively easy to make, many people do not fully appreciate the positive environmental impacts of adopting LED technology. In 2017, information provider IHS Markit estimated that the adoption of LED lighting reduced global carbon dioxide emissions that year by more than half a billion tons, or 1.5%. Life cycle assessments also indicate that compared with using incandescent or CFL bulbs, LED technology reduces hazardous waste production and risks to ecosystem and human health and better preserves air, soil, and water quality.
The future of studying the ocean lies in the development of low-cost vehicles, sensors, and instruments. These tools will enable continuous monitoring of ocean processes and allow a broader contingent of the global population to participate in ocean research and sustainability efforts. LEDs, which are useful not only for underwater lighting but also for transmitting data through water, are primed to play an important role in this future vision.
Illustration of autonomous underwater vehicles communicating data about a seafloor hydrothermal vent site.
Autonomous underwater vehicles (AUVs) communicate and work together to survey a hydrothermal vent at the bottom of the ocean in this illustration. LED lights aboard the AUVs transmit data collected through the water to receivers secured to cabled moorings. Fiberoptic cables then deliver the data from the moorings to scientists on shore, allowing them to inspect the data and, if needed, adjust their research plan in nearreal time. Credit: ©Woods Hole Oceanographic Institution, E.P. Oberlander
The oceanographic research community is currently developing fleets of autonomous underwater vehicles (AUVs) to collect physical, acoustic, chemical, and biological data that help us understand how the ocean works and how it will respond to human-induced pressures from climate change and pollution. For example, these fleets collect data that characterize (1) the changing strengths of ocean currents like the Gulf Stream; (2) the distribution and sustainability of commercially important fisheries; (3) the locations and movements of endangered marine mammals in proximity to shipping vessels and fishing gear; (4) changes in water quality parameters like pH, temperature, nutrient loads, and oxygen levels; and (5) greenhouse gas storage. These fleets also monitor the integrity of and ecosystem impacts from ocean infrastructure like installations for offshore oil exploration, wind energy production, and aquaculture.
For autonomous underwater vehicle fleets to be more than just groups of individual vehicles operating in proximity, they need to communicate and coordinate their activities. This is where advanced LED technology comes in.
In theory, these fleets will be able to take measurements across more expansive spatial and temporal scales than are currently possible while also minimizing the use of expensive and energy-intensive crewed research vessels. But for these AUV fleets to be more than just groups of individual vehicles operating in proximity, they need to communicate and coordinate their activities. This is where advanced LED technology comes in.
An estimated 436 submarine fiber-optic cables, totaling 1.3 million kilometers in length (enough for 32 trips around Earth’s equator), are currently in service, making up a complex global web of rapid-transmission communications conduits. It is this web that allows us to call friends and family nearly anywhere around the world with the touch of a button. Data are communicated through these specialty glass-lined cables in the form of high-powered light, often emitted by LEDs, that bounces from side to side in a process known as total internal reflection. Without these fiber-optic cables (i.e., if the cord, or tether, were cut), light-borne data could not travel nearly as far because the light emissions attenuate quickly.
The choice of light color makes a difference, however. In the ocean, the main attenuators of light are chromophoric dissolved organic matter (CDOM) and water itself (Figure 1). CDOM readily absorbs ultraviolet (UV) light, whereas water readily absorbs green and red light. Because blue light largely escapes absorption by CDOM and water, it travels hundreds of meters into the ocean, much deeper than UV, green, and red light. This is why the ocean is blue and why wireless underwater optical communication systems use high-powered blue LEDs to transmit data.
Diagram showing the relative importance of chromophoric dissolved organic matter and water to total light attenutation in the ocean.
Fig. 1. The relative importance of chromophoric dissolved organic matter (CDOM) and water to total light at-tenuation in the ocean is depicted here. (top) CDOM is the main attenuator of UVB, UVA, and violet light, whereas water is the main attenuator of green, yellow, orange, and red light. (bottom) Blue light penetrates deepest into the ocean because it escapes absorption by CDOM and water. Click image for larger version.
Wireless underwater LED technology is, in fact, already largely proven and in use. For example, at a seafloor borehole observatory, LED-based optical modems rapidly transmit data from in situ sensors to AUVs or remotely operated vehicles more than 100 meters away—just as similar modems did during President Faure’s livestream from Seychelles. Moreover, as seabed pipelines that deliver oil and gas from thousands of active offshore rigs to refineries on land are built and maintained, LED-based optical modems help rapidly transfer data to engineers. These data allow the engineers to monitor and react in near-real time to conditions at the seafloor, improving the accuracy and speed with which the pipelines can be laid and optimizing monitoring of pipeline health to avoid, or at least minimize, damages and environmental harm from undersea pipeline ruptures.
LEDs can also help ensure that high-quality data are collected. All sensors in the ocean—whether optical, chemical, electrical, or acoustic—are prone to biofouling or other interferences from living organisms like algal slime and barnacles that grow on sensor surfaces. Traditional approaches to minimizing biofouling involve mechanical means like wipers and shutters or chemical means like biocides. These approaches, however, leave a lot to be desired, particularly in the application of biocides that can cause collateral harm to more than just the intended organisms.
Side-by-side photos showing ocean conductivity sensors encrusted with barnacles.
Conductivity data collected using a conductivity probe deployed at sea for several months and treated with UVC light (left) matched very closely with true values, whereas data collected from a biofouled probe not treated with UVC (right) drifted away from the true values within a week [Bueley et al., 2014]. Credit: Ocean Networks Canada and AML Oceanographic
In turn, oceanographic researchers have recently been pushing for chemical-free antifouling alternatives. Given their simplicity, low costs, and power requirements, UV LEDs, particularly those that emit UVC, appear to be the wave of the future [Delgado et al., 2021], and preliminary results on their effectiveness are promising. UVC treatment has been shown to minimize biofouling of ocean sensors, improving the quality of pH, conductivity, and turbidity data collected [Bueley et al., 2014; Armstrong and Snazelle, 2017], and to allow longer deployments at sea.
Adding to the Photochemical Toolbox
From UV LEDs used for disinfection to visible light LEDs used for communications, the wavelengths generated by LEDs span the entire range of natural sunlight that reaches the sea surface. This fact opens the door for scientists to use LEDs to study how sunlight alters the cycling of major and minor elements and the fate of pollutants in the ocean, processes that influence marine ecosystems and the overall Earth system in countless ways.
Illustration of an LED-based sensor designed to determine the wavelength dependence of light-driven reactions in surface waters.
The LED-based reactor assembly illustrated here was designed to determine the wavelength (λ) dependence of light-driven reactions in surface waters that affect, for example, cycling of major and minor elements and the fate of pollutants. Wavebands range from UVB (278 nanometers) to red light (629 nanometers). A discussion of the pros and cons of this assembly compared with other technologies (related to, e.g., costs, sample throughput, portability, and data quality), as well as a step-by-step plan to build and validate an assembly, is given by Ward et al. [2021]. Credit: Reprinted with permission from Ward et al. [2021], ©2021 American Chemical Society
Aquatic photochemistry as a discipline has grown immensely over the past half century, yet in that time our understanding of how the rates of light-driven reactions depend on sunlight wavelength has not changed much. This knowledge gap matters because reaction rates of photochemical processes vary considerably from day to day, even hour to hour, and at different depths in the ocean for several reasons. First, the energy of light photons decreases with increasing light wavelength; for example, UV light is more energetic than blue light, which in turn is more energetic than red light. This variation is why sunscreen contains mineral additives that specifically block powerful, and biologically hazardous, UV light. It’s also why red pigments in street signs and artistic masterpieces fade quicker than blue pigments: More powerful blue light is absorbed by and destroys red pigments, causing visible fading, whereas absorption of less powerful red light allows blue pigments to last longer. Second, although visible light is weaker than UV light, about 10 times more visible light reaches Earth’s surface than UV light [Apell and McNeill, 2019]. Third, different light wavelengths penetrate into the ocean to different extents, with blue light penetrating the deepest.
Our limited understanding of the wavelength dependence of aquatic photochemical reactions is driven largely by cost and technological limitations. LEDs present an exciting opportunity to overcome many of these limitations.
Our limited understanding of the wavelength dependence of aquatic photochemical reactions is driven largely by cost and technological limitations. Determining wavelength dependence requires specialized equipment like high-powered lasers, solar simulators, and monochromators, which help isolate different wavelengths of the solar spectrum. This equipment is costly and energy intensive and lacks portability, often requiring researchers to stabilize or preserve their field samples for experimentation back in laboratories on land. Moreover, these approaches have low sample throughput—only one or two samples can be analyzed per day, which considerably raises labor costs.
LEDs present an exciting opportunity to overcome many of these limitations without sacrificing data quality [Ward et al., 2021], allowing a wider contingent of researchers to study rates of sunlight-driven processes over spatial and temporal scales that were previously inaccessible. Widespread adoption and application of this technology could thus allow the incorporation of photochemical processes into Earth system models and afford more accurate depictions of how major and minor elements and pollutants cycle in the upper ocean.
More People in More Places
The disruptive potential of LED technology is so broad that it is poised to play key roles in making progress toward several of the United Nations (U.N.) Sustainable Development Goals. These goals include eliminating poverty; ensuring good health and well-being for everyone; ensuring clean water supplies and adequate sanitation; fostering resilient and inclusive industry, innovation, and infrastructure; and conserving and sustainably using the oceans, seas, and marine resources.
For example, just as UVC LEDs are currently used to disinfect indoor air to combat the spread of the COVID-19-causing SARS-CoV-2 virus and other pathogens, UVC LEDs offer a low-cost and effective means to disinfect and destroy viruses and micropollutants in drinking water [Chen et al., 2017]. Moreover, visible light LEDs are a foundational technology in vertical farming, a relatively new industry that grows crops in stacked layers under controlled conditions. A key aim of vertical farming is to alleviate negative ecosystem impacts of traditional farming operations, such as reduced carbon sequestration in soils, depleted and impaired freshwater resources, and damages caused by pesticide and herbicide applications [Benke and Tomkins, 2017].
It is likely that LEDs will contribute substantially to the development of lowcost sensors to monitor ocean health, an evolution that could advance equity in the ocean sciences.
In particular, the effect of LEDs on the goal of sustainably using the oceans is becoming increasingly clear. Ocean science is global by nature, but in practice, participation is limited largely to institutions, scientists, and students in wealthy nations while being cost prohibitive in lower-income nations and for many people in ocean-dependent communities. In response to the U.N.’s call for a Decade of Ocean Science for Sustainable Development, which began in 2021, many scientists have argued for improving sustainability and equity in the ocean sciences community [Pendleton et al., 2020; Singh et al., 2021; Valauri-Orton et al., 2021; Wang, 2021]. One common recommendation in these arguments is to invest in the development of low-cost sensors and technologies to lower barriers and encourage broader participation.
Just as low-cost LED-based sensors empower air quality monitoring in developing nations that disproportionately experience the health burden of poor air quality, it is likely that LEDs will contribute substantially to the development of low-cost sensors to monitor ocean health. Such an evolution could advance equity in the ocean sciences, allowing more people in more places to participate. It could also advance sustainability by allowing us to observe and monitor more of the ocean more often, offering a wealth of information about marine conditions and health with which to make informed decisions about how we treat it.
Acknowledgments
I offer many thanks to Aleck Wang and Anna Michel for helpful discussions while I was researching this topic and to Ken Kostel, Matthew Long, Danielle Freeman, Mike Mazzotta, Taylor Nelson, Maurice Tivey, and Anna Walsh for providing constructive feedback on the article. Thanks to Katie Linehan for tolerating years of incessant conversations about how cool LEDs are. Funding was provided by the Department of Fisheries and Oceans Canada Multi-Partner Research Initiative, the National Science Foundation, and Woods Hole Oceanographic Institution.
Source: eos.org