The European Organization for Nuclear Research – known by its French acronym, CERN – is the largest particle physics laboratory in the world. Located just outside of Geneva, Switzerland, as one of post-war Europe’s first joint ventures, with the express aim of halting the ‘brain drain’ of talented scientists leaving the continent for America.
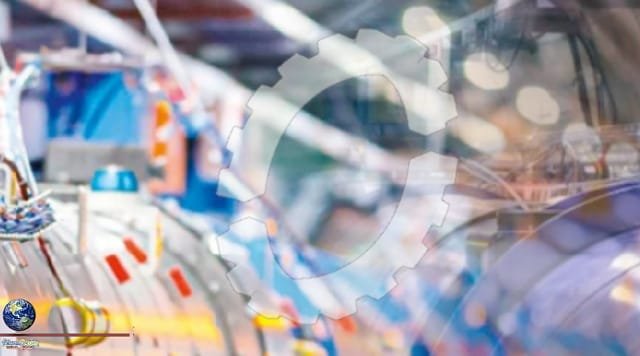
Today, more than 10,000 scientists hailing from more than 100 countries find themselves at CERN each year to use its facilities, which include some of the biggest and most complex scientific instruments ever created. Their goal: figure out what the Universe is made of and the laws of physics that dictate its behavior.
Highlights include the 1983 discovery of a pair of elementary particles called the W and Z bosons, which was later awarded the Nobel Prize for Physics. British computer scientist Tim Berners-Lee helped invent the World Wide Web at CERN in 1989 by developing a way for computers to talk to each other, called hypertext transfer protocol (HTTP).
In 1995, CERN scientists were the first to create atoms of hydrogen’s antimatter counterpart, antihydrogen. In 2000, they discovered a new state of matter: a hot, dense, particle soup called quark-gluon plasma. And the Higgs boson was observed for the first time in 2012 at CERN’s Large Hadron Collider (LHC), scooping its discoverers a Nobel Prize.
This track is an example of simulated data modelled for the CMS detector on the Large Hadron Collider (LHC) at CERN. The Higgs boson is produced in the collision of two protons at 14 TeV and quickly decays into four muons, a type of heavy electron which is not absorbed by the detector. The tracks of the other products of the collision are shown by lines and the energy deposited in the detector is shown in blue. © Lucas Taylor/ CERN
The particle collisions recreate, for a fraction of a second, the conditions that existed moments after the Big Bang, when the Universe was born. By studying the debris of these collisions, physicists try to settle mysteries such as what matter is made of and how particles get their mass.
The LHC, which was completed in 2008, was built primarily to put the Standard Model of particle physics to the test. This wildly successful theory from the 1970s describes the interactions between the 17 elementary particles and three of the four fundamental forces of the Universe: electromagnetism, the strong nuclear force and the weak nuclear force (gravity is the fourth).
An engineer works on the Compact Muon Solenoid (CMS) detector assembly in a tunnel of the Large Hadron Collider (LHC) at CERN during maintenance works
The Standard Model long predicted the existence of a never-before-seen elementary particle called the Higgs boson. After four decades of searching, in July 2012, physicists finally found it using the LHC. The discovery was a big win for fans of the Standard Model, but the theory is incomplete. It leaves many questions open, such as: what is dark matter? Why does the Universe contain more matter than antimatter? The LHC may help answer these questions.
The machine is buried deep underneath the France-Switzerland border near Geneva, in a circular tunnel nearly 27 kilometres long. It uses more than 1,000 35-tonne superconducting dipole magnets (cooled to a temperature of -271.3°C – colder than outer space!), to guide two beams of particles (usually protons) in opposite directions around the ring. The protons race around the 27-kilometre ring at almost the speed of light, completing over 11,000 laps per second.
At four points around the ring, the two opposing beams are steered so that they cross paths. Where the beams intersect, the protons within them slam into one another and shatter into smaller particles. Most of the particles produced in the collisions are highly unstable and decay into more stable forms almost instantly.
Seven enormous detectors – think of them as cathedral-sized digital cameras – are built around the four collision zones to capture data about these incredibly rare particles as they blaze briefly into existence.
The Large Hadron Collider (LHC) is the world’s largest and most powerful particle accelerator. It consists of a 27-kilometre ring of superconducting magnets with a number of accelerating structures to boost the energy of the particles along the way
The LHC was initially switched on in September 2008, with a plan to run for at least two decades. The plan European Organization includes a handful of long shutdowns where the machine is turned off so that scientists can access the equipment, perform repairs and make upgrades that allow it to operate at higher energy levels, which means more potential discoveries, during the following run.
The most recent long shutdown (LS2) began in 2019, and on 22 April 2022, the LHC has restarted European Organization after three years of maintenance work and upgrades, allowing protons to collide once again.
We spoke to Dr Monica Dunford, the physicist responsible for coordinating research on the Standard Model at the ATLAS Experiment (one of the two international collaborations credited with discovering the Higgs).
“With this next run, we’re expecting to get roughly double the total luminosity European Organization that we had by the end of Run 2,” says Dunford. Luminosity is how physicists describe the intensity of the particle beams. Doubling the luminosity doubles the likelihood of particles colliding , ATLAS announced the first-ever observation of three W bosons being produced simultaneously, from a data set taken between 2015 and 2018. Compared to creating a Higgs boson, ‘triple W’ production is about 60 times less likely to happen during proton collisions.
“It’s so rare a process that it makes us confident that maybe even in Run 3 we could possibly measure Higgs self-coupling,” says Dunford. A Higgs coupled to two others – a ‘trilinear Higgs’ – is about 2,000 times less likely than a regular Higgs.
Things could really start to get exciting after the next long shutdown, currently European Organization slated for 2026-2028. During that time, the LHC will be upgraded so heavily that it warrants a new name: the High-Luminosity LHC (HL-LHC). Over 20-plus years of operating, the machine will work up to generating luminosities nearly 30 times greater than those produced to date, allowing physicists push the Standard Model to its limits.
And the search for new physics doesn’t end there. A proposed new collider – the Future Circular Collider (FCC) – would dwarf the LHC. “It’s really just a concept right now, but ultimately this would be an even more powerful collider that would be 100 kilometres around,” says Dunford. “The LHC ring would basically just be the booster ring for the FCC!”
Source: This news is originally published by sciencefocus